Wing and Tail Aspect Ratio
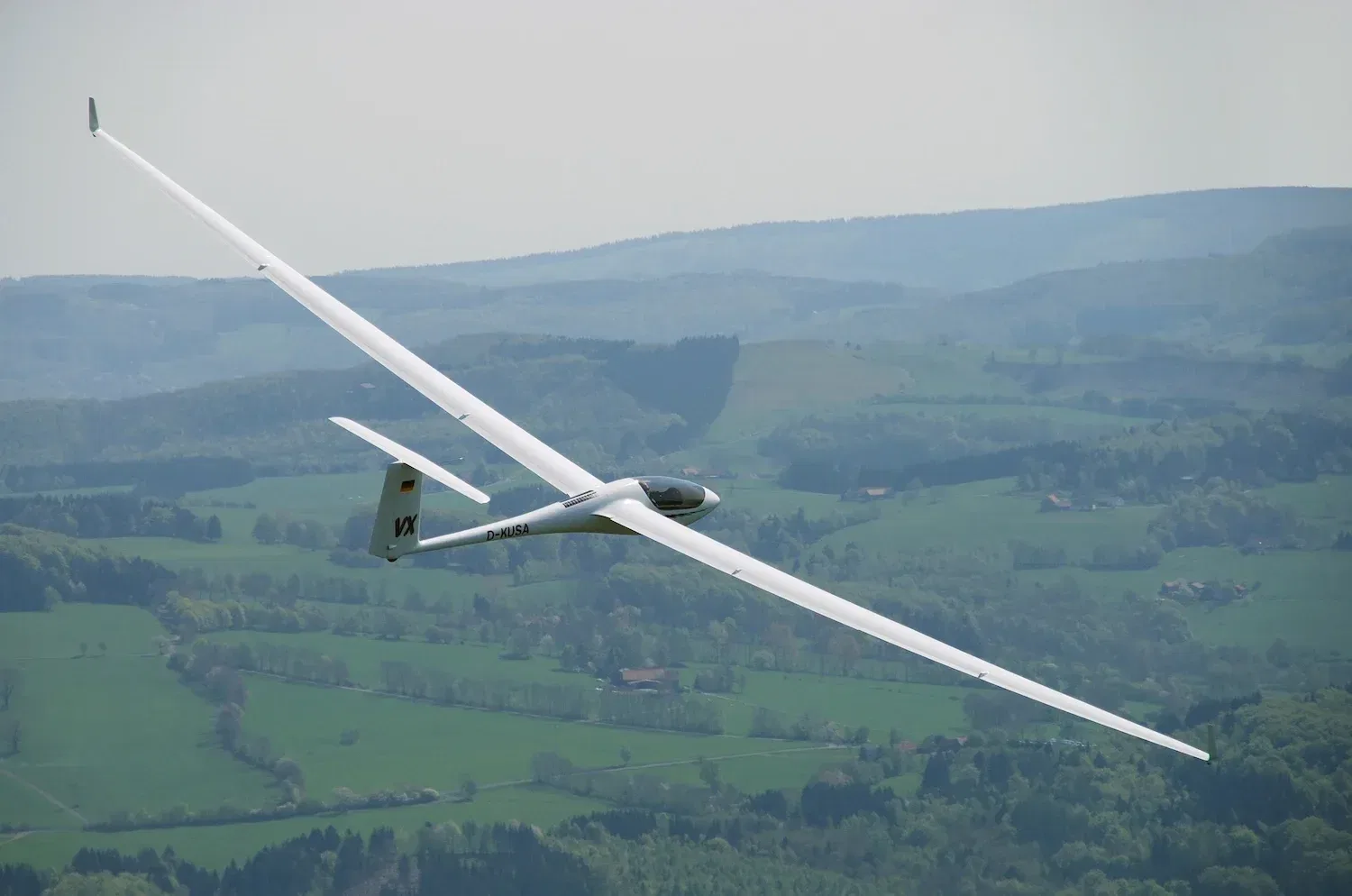
Understanding Wing and Tail Aspect Ratio in Aerodynamics
1. Introduction
Aspect ratio is a key aerodynamic parameter used to describe the shape and performance characteristics of an aircraft's wings and tail surfaces. It is defined as the square of the span divided by the surface area, or more simply, the span divided by the mean chord for rectangular wings. This ratio significantly affects the aircraft's lift, drag, stability, and maneuverability. Both wing and tail aspect ratios play distinct roles in determining how an aircraft flies.
2. Wing Aspect Ratio Calculation
The aspect ratio (AR) of a wing is calculated using the following equation:
Where:
- AR is the aspect ratio
- b is the wingspan (in meters)
- S is the wing surface area (in square meters)
For rectangular wings, the aspect ratio can also be simplified to:
Where c is the mean chord length.
Estimating Wing Surface Area: Wing surface area is typically calculated by multiplying the average chord by the wingspan. For simple rectangular or trapezoidal planforms, it can be estimated by:
- For rectangular wings
- For tapered or trapezoidal wings, a more accurate formula is
Where Croot is the root chord and Ctip is the tip chord
For more complex wing geometries, surface area may be derived from CAD models or planform integration.
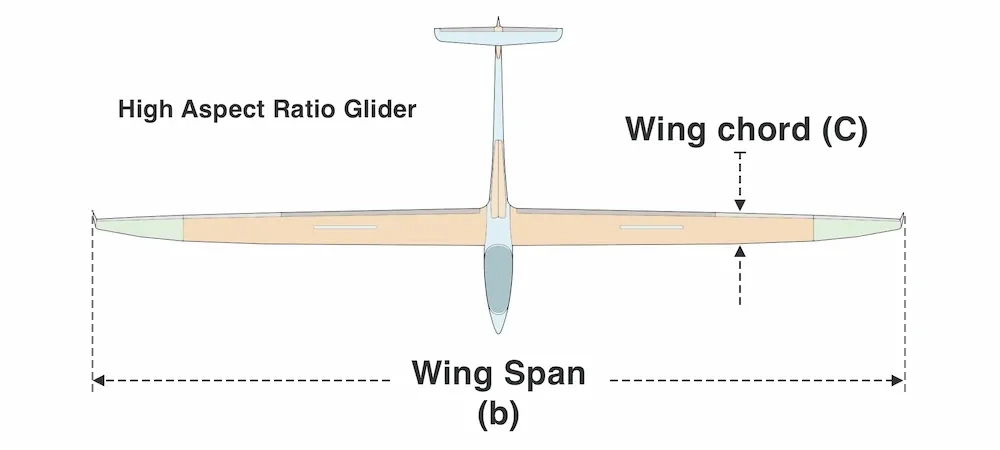
3. Wing Aspect Ratio
The aspect ratio of a wing is crucial in determining its aerodynamic efficiency:
High Aspect Ratio Wings:
- Long and narrow wings, often seen in gliders and high-altitude UAVs.
- Generate more lift with less induced drag, making them ideal for slow, efficient, and long-duration flight.
- Tend to have higher structural weight and increased bending stress.
Low Aspect Ratio Wings:
- Short and wide wings, typically used in fighter jets and aerobatic planes.
- Offer high roll rates and better maneuverability at the cost of increased induced drag.
- More robust and compact, making them suitable for high-speed operations.
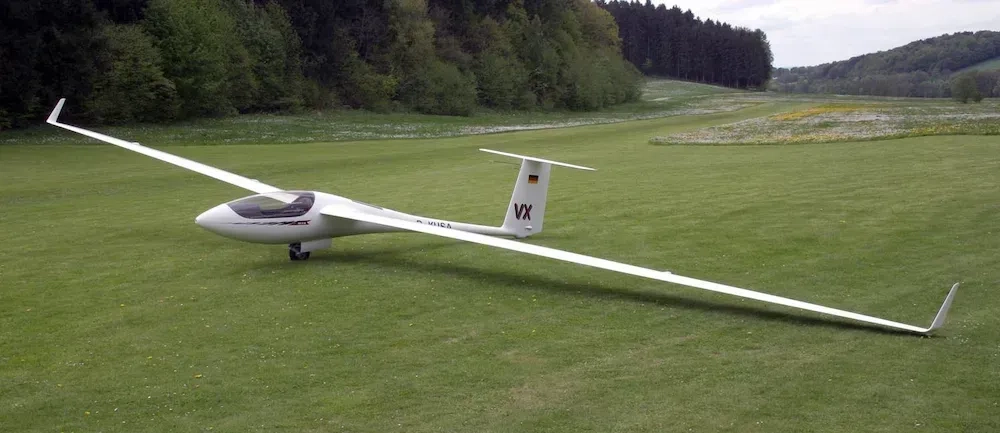
4. Tail Aspect Ratio
Tail surfaces, both horizontal and vertical, also have aspect ratios that affect flight characteristics:
High Aspect Ratio Tails:
- Provide precise pitch and yaw control.
- More effective in maintaining stability and trim.
- Common in aircraft designed for endurance and smooth handling.
Low Aspect Ratio Tails:
- Typically found in compact aircraft and jets.
- Offer structural advantages and reduce surface area.
- May sacrifice some fine control for durability and reduced drag.
Tail configuration also plays a role in determining aspect ratio efficiency. For example, T-tail and cruciform tail designs affect how the airflow interacts with the tail surfaces, which in turn influences the best aspect ratio selection for that design.
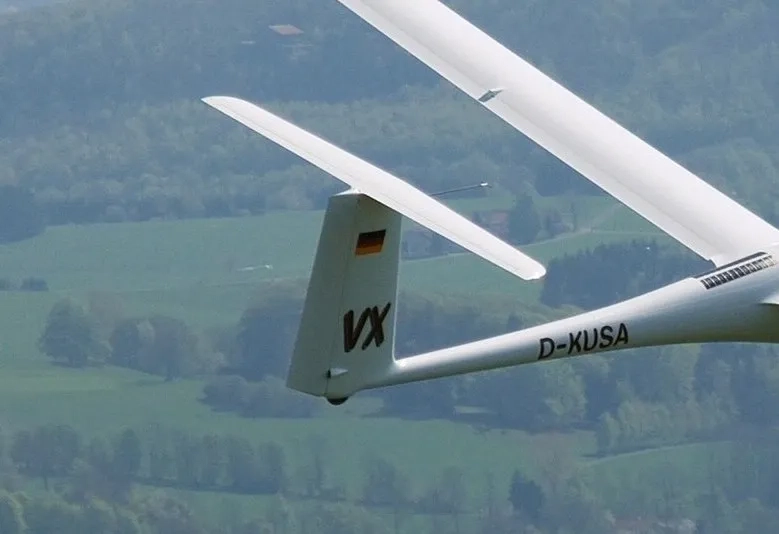
5. Flight Characteristics Affected by Aspect Ratio
- Lift-to-Drag Ratio (L/D): Higher wing aspect ratio improves L/D, which is critical for fuel efficiency and glide performance.
- Stall Behavior: High aspect ratio wings tend to stall more gradually, giving the pilot better warning and control.
- Maneuverability: Low aspect ratio wings respond faster to control inputs, essential for combat or stunt maneuvers.
- Stability: Tail aspect ratio influences pitch and yaw damping, affecting how stable and responsive the aircraft feels.
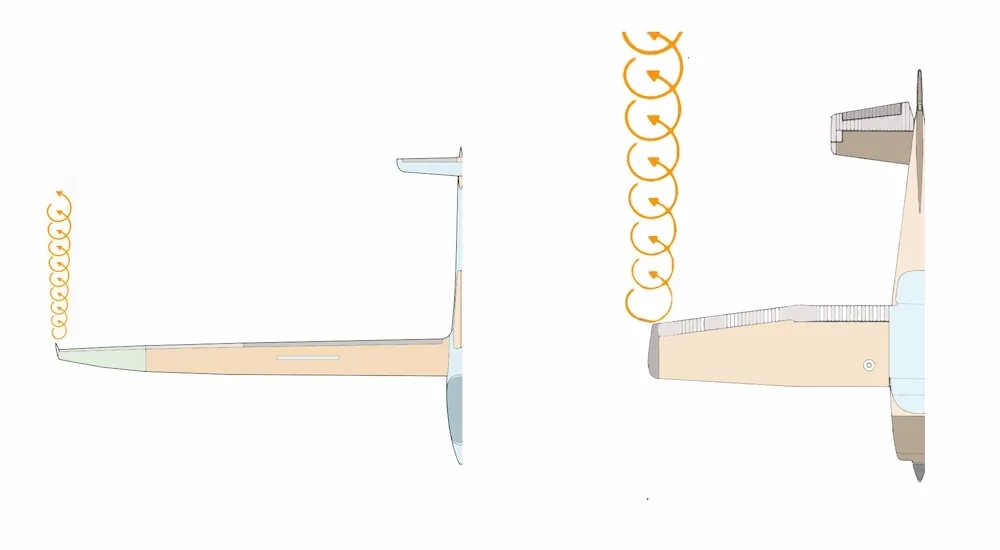
6. Additional Design Considerations
While high aspect ratio wings offer aerodynamic advantages, such as better lift-to-drag ratios, not all aircraft are designed with them due to a range of engineering and operational tradeoffs:
Structural Challenges: Long wings experience higher bending stresses under load, requiring stronger and often heavier structures. They are also more prone to torsional deformation, which can interfere with control surfaces like ailerons.
Maneuverability: Low aspect ratio wings have lower moments of inertia, allowing faster roll rates—an essential feature in fighter aircraft. Additionally, shorter wings support longer chords and thinner airfoils, which are beneficial for supersonic flight.
Parasitic Drag Considerations: Although high aspect ratio wings reduce induced drag, they can have slightly higher parasitic drag. For equal wing areas, a high aspect ratio wing has a smaller chord. Due to Reynolds number effects, the section drag coefficient increases slightly as chord length decreases. This increase is modest compared to the gains from reduced induced drag. For example, a 20 percent increase in chord length may reduce the section drag coefficient by about 2.4 percent.
Internal Volume and Systems: Low aspect ratio wings generally have a thicker cross-section and longer chord, which provides more usable internal volume for fuel tanks, landing gear, and other systems.
Airfield Constraints: Aircraft wingspans are limited by airport infrastructure, including runway widths, taxiways, and hangars. Designers sometimes reduce aspect ratio to keep the wingspan within operational limits while increasing the wing area via chord length. For instance, the Airbus A380 is limited to an 80-meter wingspan with an aspect ratio of 7.8, while aircraft like the Boeing 787 or Airbus A350 achieve aspect ratios closer to 9.5.
7. Real-World Examples
- Schleicher ASH 31 Glider
- Wingspan: 21.0 meters
- Wing Area: 12.6 square meters
- Aspect Ratio: 35.0
- Lift-to-Drag Ratio: approximately 56:1
- Aircraft Weight: 525 kg (maximum)
- Wing Loading: 41.7 kg/m²
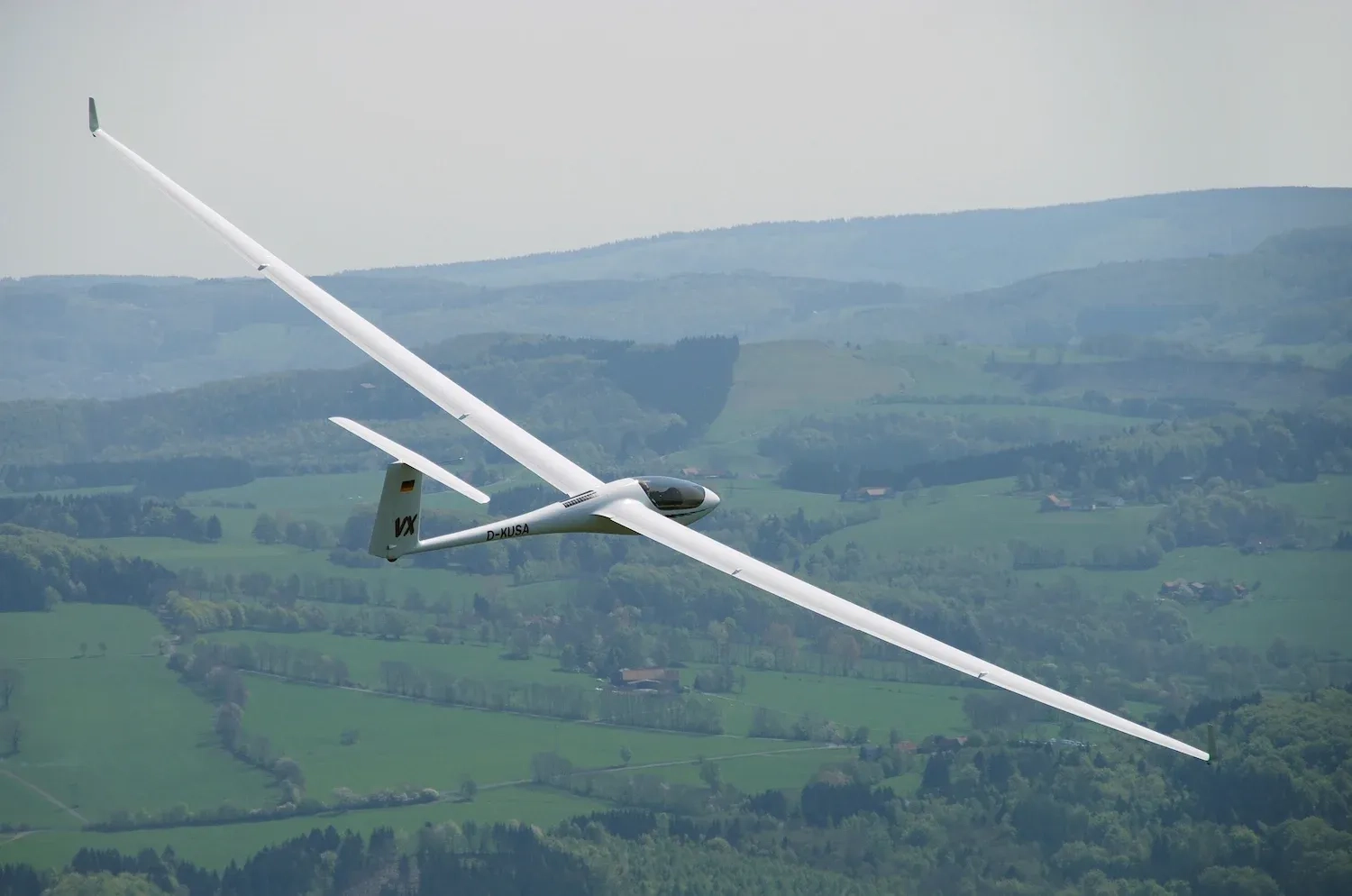
- Cessna 182M Skylane
- Wingspan: 10.97 meters
- Wing Area: 16.2 square meters
- Aspect Ratio: 7.4
- Lift-to-Drag Ratio: approximately 13:1
- Aircraft Weight: 1250 kg (maximum takeoff weight)
- Wing Loading: 77.2 kg/m²
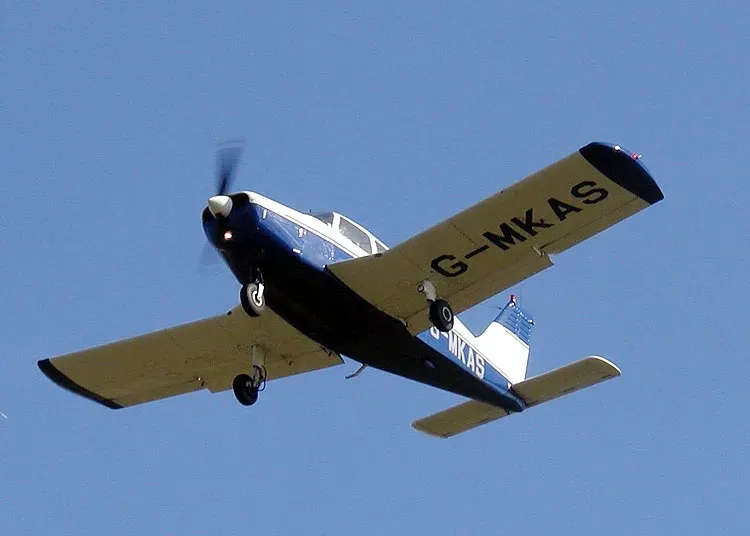
- F-16 Fighting Falcon
- Wingspan: 9.96 meters
- Wing Area: 27.87 square meters
- Aspect Ratio: 3.6
- Lift-to-Drag Ratio: approximately 5.0 (varies with speed and configuration)
- Aircraft Weight: 12000 kg (clean configuration)
- Wing Loading: 430.6 kg/m²
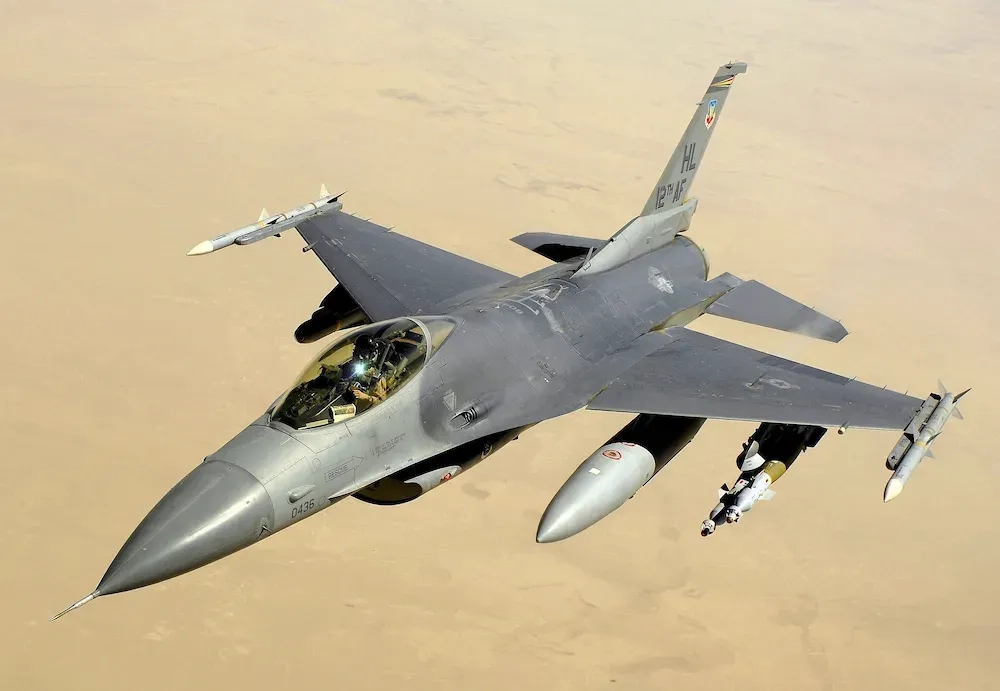
8. Summary
Aspect ratio is a fundamental design variable in aircraft engineering. High aspect ratios have aerodynamic efficiency and stable flight characteristics, while low aspect ratios enhance maneuverability, structural practicality, and internal space. Engineers must balance these tradeoffs based on the aircraft's intended mission, performance goals, and physical constraints.
By understanding the influence of both wing and tail aspect ratios, designers can optimize aircraft for a variety of roles—from high-efficiency gliders to agile supersonic jets.